Newsletter #7: From bench to bed side: how the new preclinical stroke models change the game.
Strokes are the second most common cause of mortality in the over-60s and the leading cause of acquired disability. There are currently only two approved treatments for the management of patients affected by acute ischemic stroke (AIS): 1) Intravenous administration of a fibrinolytic drug (recombinant tissue plasminogen activator (rt-PA, Alteplase)) and 2) Thrombectomy – surgical intervention allowing mechanical removal of the clot.
Therapeutic options remain inadequate, since only around 20% of patients in Western countries are eligible for one of these treatments, and even those have relatively limited effectiveness. For patients treated with Alteplase, a total reperfusion at 24 hours is observed in only 50% of cases – and more than 40% of patients are either left with severe disability, or die.
Thrombectomy allows reperfusion in 90% of cases, but around 40% of patients presenting a modified Rankin Scale score of 2-3 die within 90 days of the AIS.
There is an urgent need to improve the prevention and treatment of strokes, the incidence of which is certain to increase as the population ages: the authors of the Stroke Action Plan 2018-2030 highlight an expected rise of 35% in the number of people at risk by 2050 as describe in Newsletter #5.
For the past 20 years, despite tests on more than a million molecules using different therapeutic strategies and targets aimed at improving the effectiveness and safety of recanalization, protecting the brain and promoting recovery, no new drug has demonstrated clinical effectiveness.
The suitability of the animal models used for evaluating and selecting these drug candidates has been widely called into question. After 15 years of hard work, lessons can be drawn from these failures – and new, more translational tools are available.
In January 2021, Strok@lliance‘s academic partner, the Physiopathology and Imaging of Neurological Disorders (PhIND, UniCaen) team led by Professor Denis Vivien, published a review entitled “Filling the gaps on stroke research: focus on inflammation and immunity” in the journal Brain, Behaviour and Immunity. It examines recent examples of strategies targeting neuroinflammation in stroke and highlights weak points in the transition from the preclinical to the clinical phase.
In order to illustrate the importance of choice of preclinical model in drug discovery strategies, we will summarise some of the main points of the review here.
First we will provide some background facts on neuroinflammation (NI) and immune response (IR) following an AIS. Next, we will cover the clinical results obtained for certain molecules that target NI and IR. Finally we will move on to a critical analysis of the animal models that have shown these molecules to be effective, and of their consistency with the results of clinical studies.
1. Immune response in the physiopathology of stroke
The general framework of the physiopathology of stroke has been well defined in recent decades. The consequences of ischaemia, decreases in glucose and oxygen supply, the appearance of oedema and neuronal death, the progression of the initial lesion linked to oxidative stress, excitotoxicity and microvascular lesions are already described well in the literature. In recent years, the role and mechanisms of neuroinflammation and the peripheral immune response have been the subject of particular interest, which has led to the evaluation of several drug candidates in clinical trials.
What do we know? The immune response happens in the first minutes following the AIS: ionic imbalances and a lack of nutrients quickly trigger activation of the microglia. Damage to the ischaemic tissue amplifies this activation, in particular through the release of purines and damage-associated molecular patterns (DAMPs): heat shock proteins, high mobility group box and interleukin-33, peroxiredoxins, mitochondrial-derived N-Formyl peptides. The activated microglial cells will then release pro-inflammatory cytokines (including IL-1Beta) in the ischaemic core and the penumbra. This activation will also spread to other types of cells such as astrocytes, and later endothelial cells (ECs).
The integrity of the blood brain barrier (BBB) is gradually compromised, allowing DAMPs and cytokines to move out of the Central Nervous System (CNS) towards the bloodstream. Immune cells are then recruited in the region of the arterial endothelium. The leukocytes – that is, lymphocytes, neutrophils or macrophages – thus recruited will interact in particular with integrins expressed by the ECs (through the leukocyte rolling mechanism) and subsequently migrate from the blood compartment towards the brain tissue. The main adhesion molecules involved here are: ICAM-1, VCAM-1 and P-selectin. Once introduced into the brain tissue, leukocytes will in turn produce pro-inflammatory agents which will further increase the immune response. This is how neutrophils, for example, intensify the recruitment of other lymphocytes.
These neutrophils will also worsen the initial damage by clogging microvessels, damaging ECs and degrading the extracellular matrix. These effects can result in the formation of secondary thrombi, rich in platelets.
All of these processes maintain and amplify each other, leading to a surge in the immune response which reaches its peak in the 24 to 48 hours following the AIS. This generalised description should be modified according to the location in the brain of the ischaemia, as shown in Fig. 1.
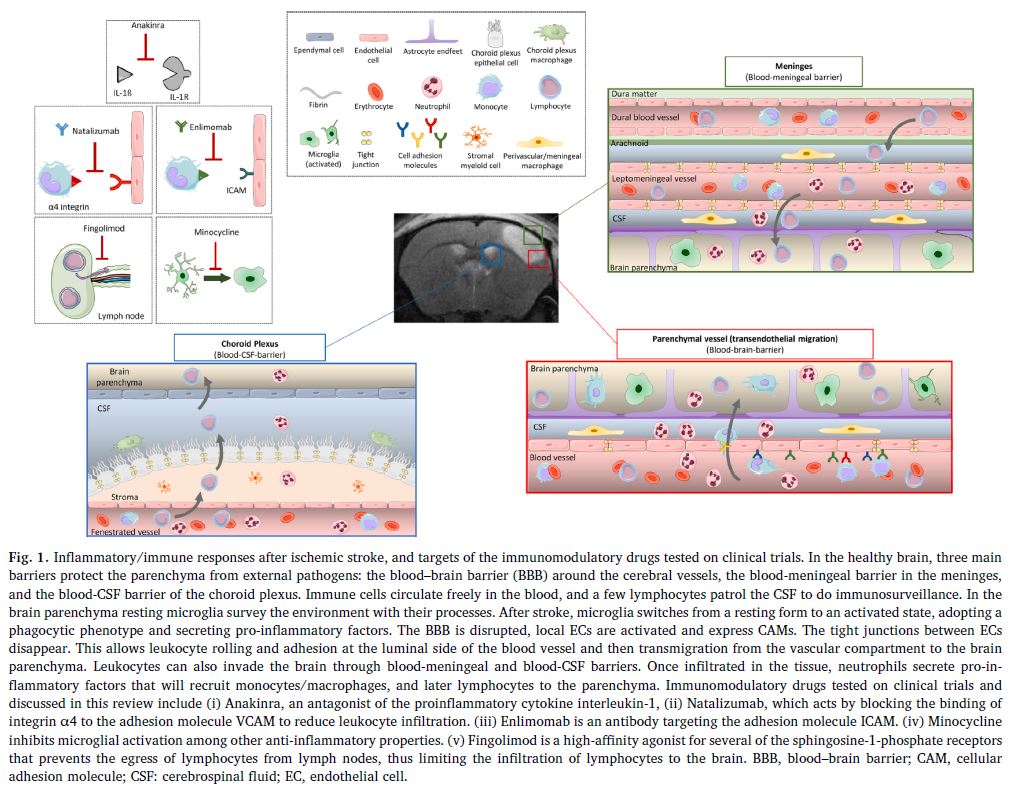
After this acute phase, the immune response gradually diminishes and gives way to processes that eliminate cellular debris, with the release of anti-inflammatory agents and pro-survival factors. This phase allows tissue to regain its integrity and brings about the restructuring of the matrix, neurogenesis, axonal routing, dendritogenesis and oligodendrogenesis.
At the same time we observe a systemic immunodepression which makes patients more vulnerable to lung and urinary infections, a complication of AVC observed in 20% of patients.
The importance of these inflammatory responses in the advancement of the ischaemic damage, the rupture of the BBB and the formation of the oedema have made it a target of interest for pharmacological approaches. Unsurprisingly, these approaches target the acute phase of the immune response, with the aim of limiting the stages of activation of the microglia and the recruitment of leukocytes.
1. Results of the main clinical trials targeting neuroinflammation
Several drugs aimed at reducing the effects of neuroinflammation and the immune response following an AIS have already been the subject of clinical assessments.
a. Anakinra
Anakinra is a drug which has drug marketing approval for the treatment of rheumatoid arthritis. Its capacity for acting as an antagonist in the interaction of IL-1beta with its receptor (IL-Ra) makes it a potential candidate for the treatment of neuroinflammation following an AIS. In clinical trials, Anakinra significantly reduced the level of IL-6 and C-reactive protein, both of which are markers of inflammation. However, 90 days after stroke onset, no change to the modified Rankin Scale was observed in patients treated with it compared to the control group. Nevertheless, this approach is still open to further exploration, since a negative reaction with rt-PA could have disguised its efficacy.
b. Minocycline
Minocycline, a tetracycline derivative, blocks the activation of the microglia and iNOS and reduces the excitotoxicity linked to glutamate. A pilot clinical study researching the effective dose of Minocycline administered intravenously showed no harmful effect in co-administration with Alteplase, though no beneficial effect of the combination was observed either. However, a metanalysis of other randomized controlled trials (RCTs) including those using other means of administration showed a promising neuroprotector effect of this molecule in AIS.
c. Natalizumab
Natalizumab is already used for the treatment of auto-immune conditions such as multiple sclerosis and Crohn’s disease; this antibody targeting alpha-4-integrin prevents its interaction with VCAM-1, and therefore the infiltration of leukocytes in the CNS.
Although an initial clinical trial showed global positive effects on the Stroke Clinical Impact-16 and on cognitive function at 90 days, no reduction in the volume of the lesion was shown in patients treated with Natalizumab. These encouraging results were not found in subsequent studies, particularly in trials in combination with a thrombolytic treatment or a thrombectomy.
The clinical development of Natalizumab indicated for stroke was stopped in phase IIb.
e. Enlimomab
Enlimomab is an antibody targeting ICAM-1, it also enables the reduction of infiltrations of leukocytes in the CNS. Despite positive preclinical trials, an increase in mortality in patients treated during phase III led to the cancellation of clinical trials of this molecule.
f. Fingolimod
Fingolimod is an immunosuppressant with drug marketing approval for the treatment of multiple sclerosis. It binds to sphingosine 1-phosphate (S1P) lymphocyte receptors and thus directly limits their recruitment to the lymph nodes. Clinical trials assessing its efficacy have brought to light a significant beneficial effect on the advancement of brain damage in the penumbra, the alleviation of neurologic deficits and the acceleration of recovery with a significant improvement of the modified Rankin Scale at 90 days post-AIS.
It is certain that all of these molecules tested in clinical trials would present promising results during phases of preclinical development, with an acceptable safety profile. What lessons can we draw from these trials in order to improve preclinical research?
2. The contribution of preclinical studies: the example of stroke models of middle cerebral artery occlusion (MCAO)
Ever since 1999 and the first Stroke Therapy Academic Industry Roundtable (STAIR) recommendations, the research community in the field of stroke has agreed on the need to use reproducible models, applied according to rigorous methodologies that meet clinical standards (blind trials, randomised, statistical power taken into account, transparency over data, a priori assessment and exclusion criteria, etc.) with the aim – even 20 years ago – of improving the predictiveness of preclinical trials.
Despite these recommendations (which have been updated several times) and the application of increasingly sophisticated methodological precautions, it has to be said that to date, no product has demonstrated its efficacy in a clinical setting. Besides the methodological problems, it is indeed, therefore, the intrinsic relevance of animal models – particularly those using rodents – that must be called into question.
In his article, Professor Vivien’s team show that several of the most frequently used rodent models are only very weakly representative of human physiopathology in relation to neuroinflammation. Recent improvement in in vivo molecular imagery allows for better characterisation of the kinetics of inflammation in the most widespread rodent models.
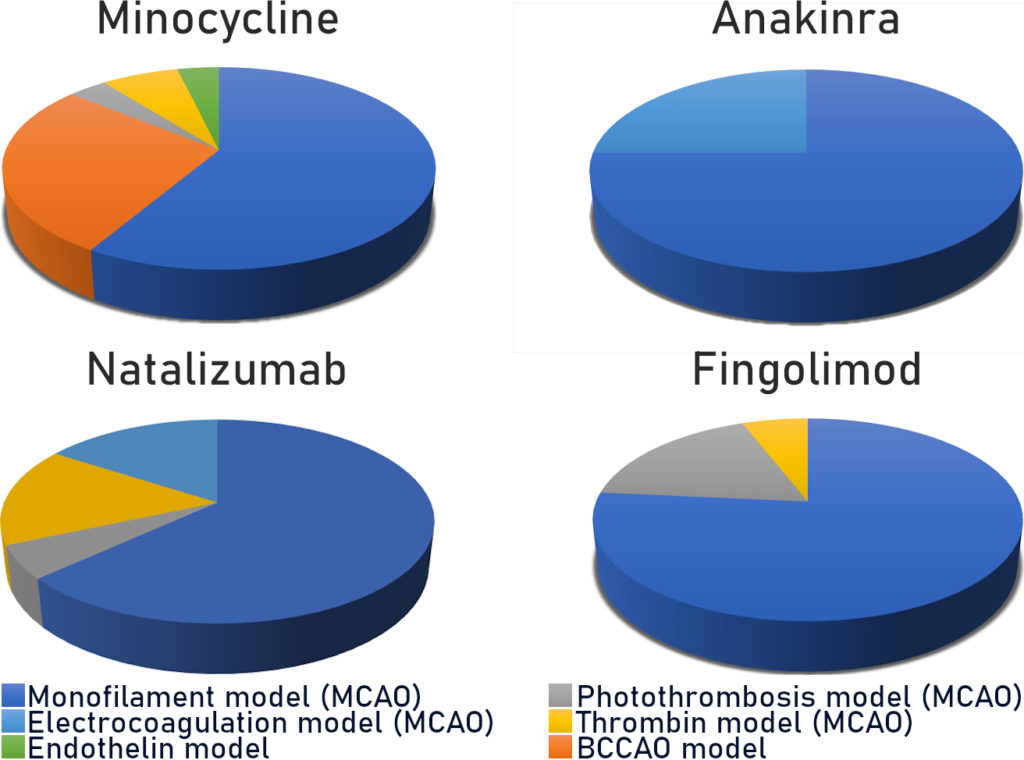
a. Model of mechanical MCAO by intraluminal filament (monofilament model)
This “gold standard” model relates to 60 to 75% (Fig. 2) of studies carried out using rodents. As a reminder, this model consists of mechanically occluding the Middle Cerebral Artery (MCA) with a filament inserted via the internal carotid. This model is well calibrated and controlled. It is reasonably reproducible and produces fairly consistent results. However, recent characterisation studies of this model have shown that the sudden reperfusion at the moment of the filament removal, the intensity and speed of the onset of the inflammatory reaction as well as the huge, rapid, rupture of the BBB are not representative of an AIS in humans (Tab. 1).
Thus, in this model, all the anti-inflammatories tested, i.e. Natalizumab, Fingolimod, Anakinra and Minocycline, presented positive effects which were unrelated to their clinical efficacy.
Since this model has already produced thousands of false positive results and presents other differences with human pathology (cf. Expert Opinion – Ischemia-reperfusion injury- Recent clinical data challenge the translational value of mechanical MCAO models of stroke in rodents ), it should not be used without an excellent reason for doing so (Tab. 2).
b. Photothrombosis model
The second most widespread model is photothrombosis (Fig. 2). This consists of injecting a photosensitive dye into the bloodstream to be activated by light locally. This model allows the inducement of a very precise and local ischaemia due to the formation of a clot rich in platelets. Characterisation studies have also shown that the activation of the photosensitive dye produces a significant local toxicity caused by the production of free radicals. These particularly affect endothelial cells and bring about a significant and rapid rupture in the BBB, thus facilitating the onset of a hyperintense inflammatory response. Moreover, in this model there is no penumbra, and pharmacological or mechanical recanalization of the tissue is difficult to achieve: two major divergencies from human pathology (Tab. 1)
With regard to the molecules tested in this model: Natalizumab, Fingolimod and Minocycline, have all shown positive effects on the volume of damage, which has not been confirmed by the RCTs for Natalizumab (Tab. 2).
c. Electrocoagulation model
Use of the MCA electrocoagulation model is also quite widespread in stroke studies. This model entails using an electric current to induce coagulation of the MCA. It therefore enables the production of an ischaemia without clotting, without possible reperfusion and without a penumbra. In this model a disproportionate immune response with regard to AIS is observed, with a massive infiltration of leukocyte in the acute phase.
In terms of the predictive value of the model, effects on the reduction of the volume of the lesion have been observed for Natalizumab but these have not been confirmed in RCTs.
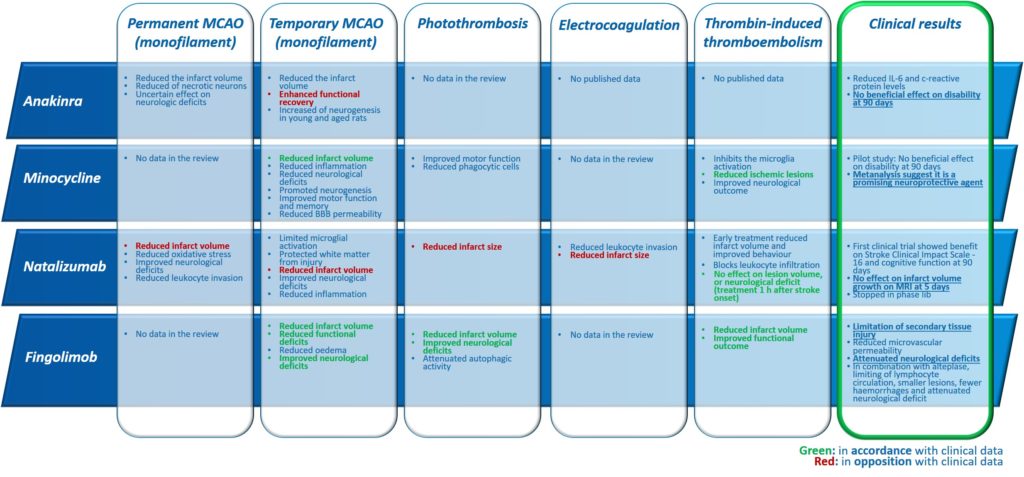
d. Thromboembolic model
The final model which interests us here is a less widespread model of thromboembolism induced by injection of thrombin directly into the M2 segment of the MCA. The injection of thrombin causes the formation of a clot rich in fibrine which, as in the clinical setting, can spontaneously undergo lysis. This model is sensitive to the fibrinolytics effects of the rt-PA and has a short therapeutic window. Beyond this window, the risk-benefit balance disappears, as it does in humans. The rt-PA limits brain damage, particularly by reducing damage to the penumbra zone. This model presents a temporal dynamic for immune response comparable to that observed in humans, in particular a gradual leucocyte infiltration with a peak at 24 hours post-ischaemia, as well as an activation of the endothelial cells and a gradual opening of the BBB (Tab. 1, Drieux et al 2020).
These similarities with human pathology appear to give it a good level of predictivity, at least for the evaluation of molecules targeting inflammation. In this model, Natalizumab did not show beneficial effects, whereas Minocycline presented beneficial effects consistent with clinical observations. Other studies should allow conclusions to be made about the predictivity of the model in assessing other drug candidate molecules (Tab. 2).
This model also offers many advantages for experimental pharmacology applications. In comparison with the clinical situation in humans, the thromboembolic model has broad similarities in terms of:
- Volume of infarcted tissue: in the thromboembolic model, the lacunar stroke produces only a 10% lesion of the ischemic hemisphere, whereas in the monofilament model, lesion volumes represent 30-40% of the affected tissue (Fig 3). The thromboembolic model, then, induces medium-sized lesions, such as those observed in patients included in the RCTs. Indeed, patients with large lesion volumes following a massive stroke are generally excluded from RCTs.
- Embolism location: the thromboembolic model targets segment M2 of the MCA, whereas other models induce a more proximal occlusion located at the base of the MCA. It is important to note that proximal clots are only slightly sensitive to the effect of rt-PA, and thus have a low rate of recanalization, unlike distal clots (M2 segment Fig. 3). Since proximal clots can be removed by thrombectomy, it is suggested that monofilament-induced ischemia/reperfusion could be considered as a thrombectomy model. However this is not the case as discussed in our Expert Opinion – Ischemia-reperfusion injury: Recent clinical data challenge the translational value of mechanical MCAO models of stroke in rodents
- Time course of stroke pathophysiology:
- total or partial spontaneous reperfusion is observed within the first 24 hours of stroke onset
- gradual disruption of the BBB
- inflammatory response close to that of patients: leucocyte recruitment is gradual, peaking 24h post-stroke and visible for 5 days
- activation of endothelial cells from 24-48h to 5 days post-stroke
- maximum lesion size reached at 24-48h post-stroke
- Time course of treatment efficacy:
- The longer treatment is delayed after the stroke has occurred, the greater the damage will be
- Recanalization is gradual, whether spontaneous or pharmacological
In comparison with other MCAO models, there are also other methodological advantages:
- Lower mortality rate: the thromboembolic model induces mortality of about 1%, compared to the monofilament model which results in 30-40% mortality.
- Lesions that are both reproducible and representative: In the monofilament model, we observe cortical damage as well as lesions in the hypothalamic region, hippocampus or striatum. In the thromboembolic model no lesions are observed in other regions or outside of MCA territory. This specificity significantly reduces the list of exclusion criteria relating to “abnormal lesion patterns” or “unachieved occlusion”.
- Comparison (or possible combination) with a standard treatment (rt-PA)
All the above-mentioned points facilitate analysis of the data, avoiding interpretation bias in relation to the inclusion of animals and mortality rates. In this way, they can be used to ensure unambiguous conclusions.
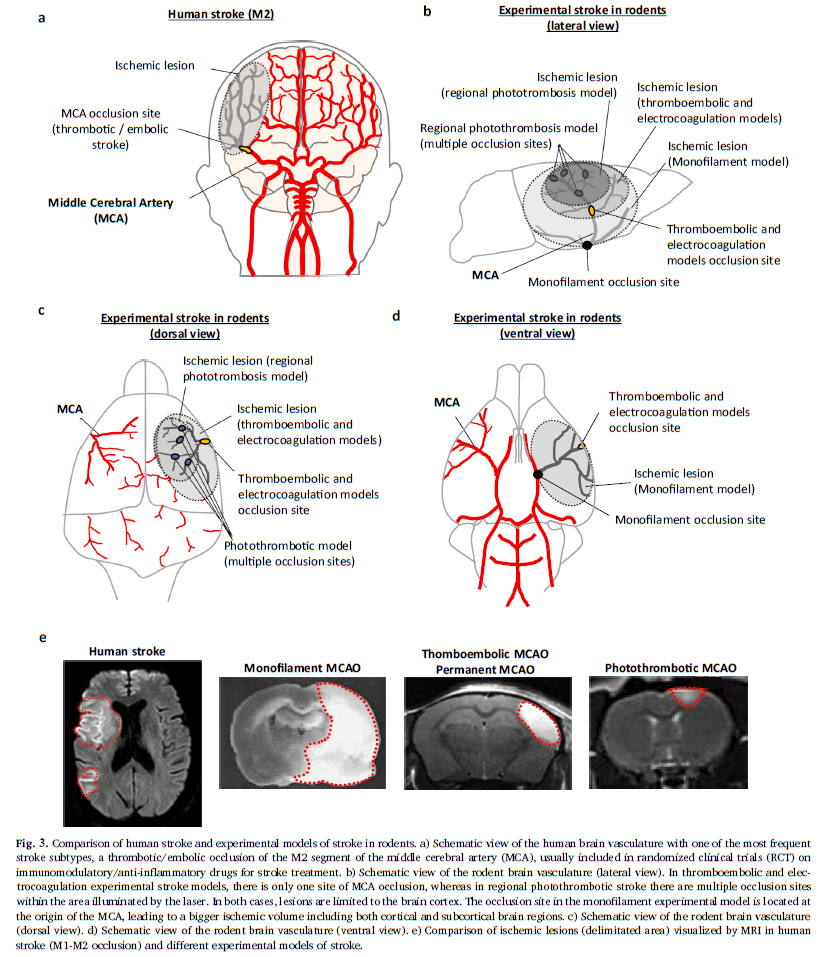
3. Conclusion
All the molecules which target stroke which were tested in a clinical setting showed positive effects in preclinical studies, particularly in the monofilament model, but then mostly ended up failing in clinical trials. The lack of selectiveness in classic models – the monofilament model in particular – cannot be explained by methodological problems alone. In the case of neuroinflammation, this means that the physiopathological processes it brings about correspond poorly to human pathology.
The use of more relevant animal models from a clinical point of view, such as the thromboembolic model proposed by the Strok@lliance, appear to be essential in improving the predictivity of preclinical trials. Moreover, the results of several suitable models should be systematically compared and cross-referenced, in order to ensure a better selection of candidates for clinical studies.
For more information, or for scientific advice about your preclinical studies, our experts are here, ready to answer your queries.